Pathways of Electron Transfer
NREL researchers aim to understand the fundamental properties of the inorganic and organic cofactors that mediate electron flow in proteins and how the properties are tuned by the chemistry of protein complexes.
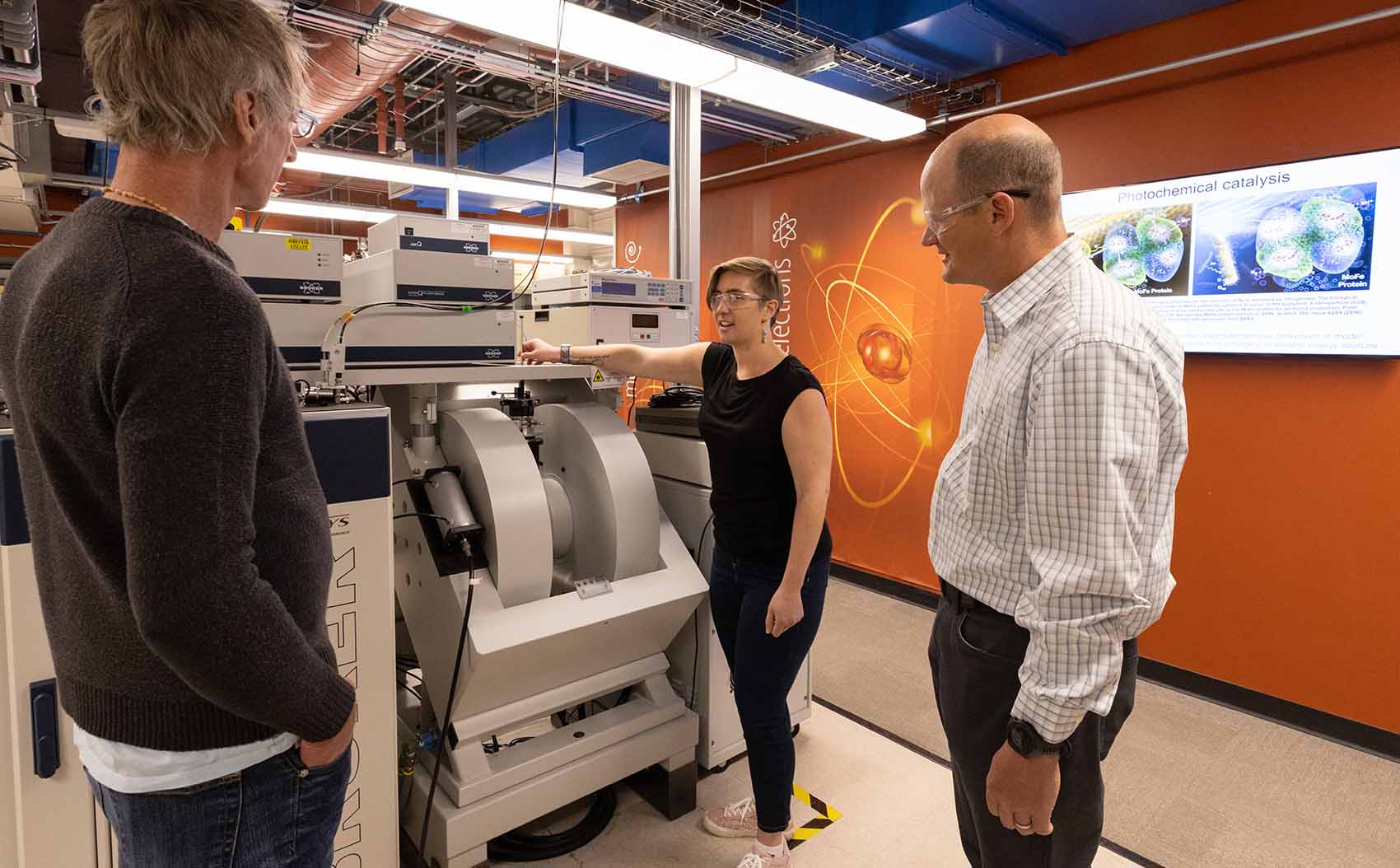
Photosynthetic Energy Transduction
Natural photosynthesis functions across an expansive range of environments, from aquatic to microbial mats to land. We aim to elucidate how plasticity in reaction centers and enzyme structures enables control of the photochemical, electron transfer, and catalytic reactions that are essential to transformation of solar energy into reduced compounds and chemical products. Resolving the fundamental operating principles of photosynthesis provides foundational knowledge to guide the design of efficient artificial solar energy conversion systems.
Electron Bifurcation
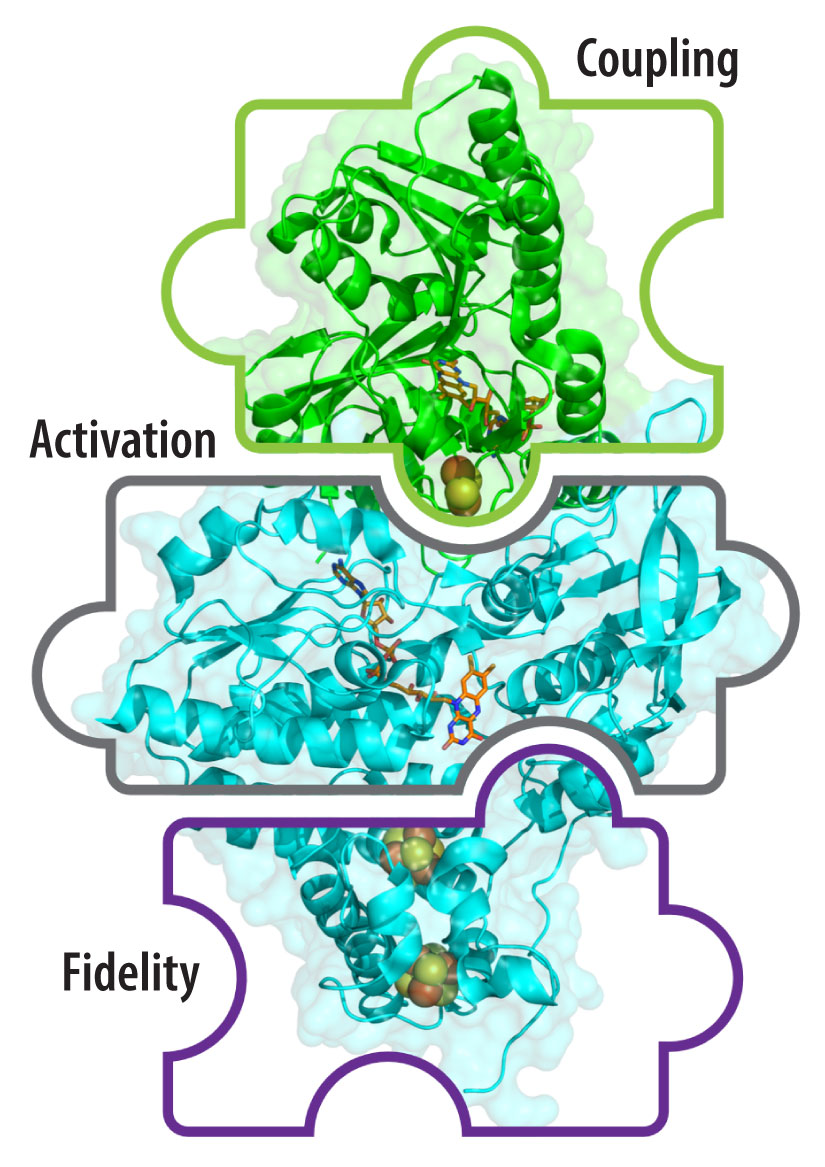
NREL researchers are uncovering the mechanistic determinants of enzymatic electron bifurcation, a remarkably energy-efficient process employed by various microorganisms to ensure their survival in harsh, energy-limiting environments. During bifurcation, an electron pair from a medium-energy two-electron donor is split apart, with each electron transferred down spatially and energetically distinct pathways. The result is a product with higher energy than the starting material, and another with lower energy. Understanding the mechanistic underpinnings of electron bifurcation could enable breakthroughs in our ability to create valuable, high-energy products from lower-energy inputs.
Our team explores the intricacies of the bifurcation mechanisms in the NADH-dependent reduced ferredoxin: NADP+ oxidoreductase I from Pyrococcus furiosus, the Nfn-BfuABC complex from Thermococcus sibiricus, and the [FeFe]-BfuABC from Thermotoga maritima.
To learn more, read how NREL Research Dives Deeper Into the Mysteries of Energy Control in Electron-Bifurcating Enzymes.
Contact: Cara Lubner
Role of Electron Spin in Biological Electron Transfer and Catalysis
NREL researchers are focused on understanding the role of electron spin in multi-electron redox catalysis. Electron spin, the intrinsic angular momentum of the electron, is fundamental to the structure and interaction of metal sites and catalytic cofactors that form the redox circuitry of enzymes.
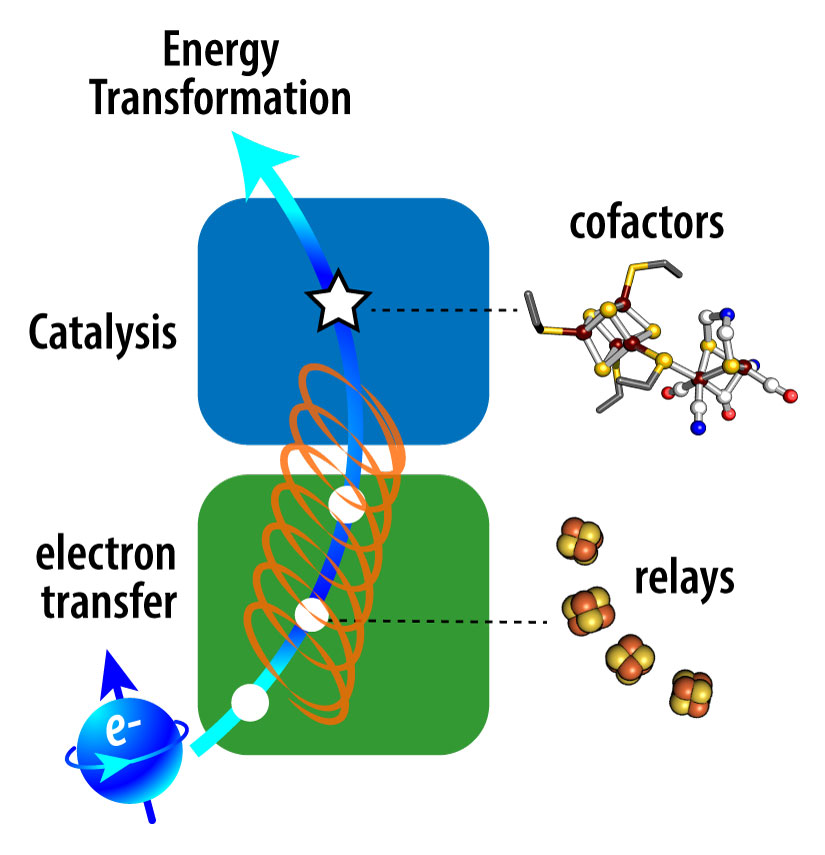
Alignment of electron spin is sensitive to its environment and can be modulated by chiral molecules. From photosynthetic electron transfer to the oxygen evolution reaction, the conservation, selectivity, and modulation of spin underpins biological energy transformation processes. We are addressing knowledge gaps for how the mechanisms of transfer and chemical conversion are tuned by spin-dependent properties of iron-sulfur clusters that comprise electron transfer relays and catalytic cofactors found in redox enzymes.
Fundamental understanding of how protein structures and interactions have evolved to control spin is expected to lead to new strategies to enhance efficiency and control over the selectivity of multi-electron chemical reactions in biomimetic and engineered systems.
Contact: David Mulder
Flagship Projects
NREL researchers advance understanding of molecular mechanisms that govern energy capture and conversion reactions important to photosynthesis. We seek to design systems with the ability to synchronize reaction networks under dynamic fluctuations in solar irradiance and reaction rates. Our research addresses gaps in the understanding of how reaction components and pathways respond to variations in energy flux.
Our strategic research objectives are to elucidate the mechanistic basis of PSI plasticity in modulating the photochemical and light harvesting properties in response to energy imbalance and develop a deeper understanding of the properties and mechanisms of redox enzymes for coupling electron and proton transfer to reactivity with ferredoxin, NAD(P)(H), H2 and O2. Emerging research addresses the role of spin in the control of enzyme reactivity and the compositional variations in PSI and phycobilisomes from strains with altered electron utilization.
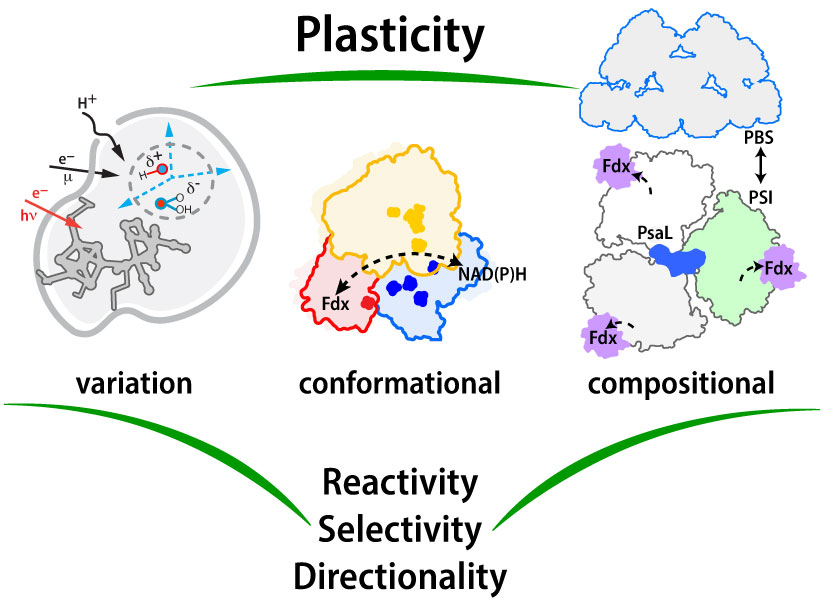
Pathways of Electron Transfer Core Program Vision—To elucidate how structural plasticity in the molecular components of photosynthesis govern the mechanisms and efficiencies of energy transformation reactions. Image by David Mulder, NREL
Contact: Paul King
NREL researchers use an assembly of nanocrystal-nitrogenase biohybrids as a model system to elucidate how solar energy is used to convert dinitrogen (N2) into ammonia (NH3). The biohybrid system is a unique reporter for understanding the component properties and system parameters that govern the photochemical kinetics and efficiencies of the N2 reduction reaction.
We aim to understand the nanocrystal properties that are essential for electron delivery and reactivity with nitrogenase, elucidate the nanocrystal-nitrogenase interactions that stabilize binding and the function of binding site in electron delivery, use time-resolved approaches to develop kinetic models, and identify rate limiting steps in the photochemical reaction. Our approaches include dissecting the effects of excitation rates and hole scavengers on substrate selectivity and photochemical NH3 production reaction rates. We use time-resolved spectroscopy to measure the effects of nanocrystal properties on electron transfer and hole-transfer kinetics, and electron paramagnetic resonance spectroscopy of biohybrids under illumination in the frozen state to enable quantification of intermediates in the pre-steady state.
The results of these studies are being simulated by chemical kinetic and Monte Carlo kinetic models to gain insights on the reaction parameters and rate constants and an understanding of factors controlling ammonia production efficiencies and yields.
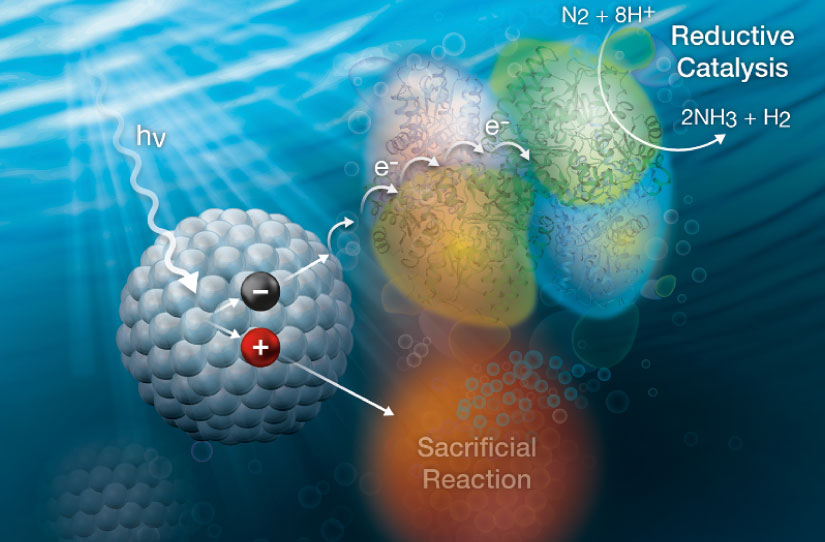
Our vision is to understand the physical, kinetic, and thermodynamic principles that control the coupling of nanomaterial photochemistry to nitrogenase catalysis and photochemical reduction of N2 to ammonia. Image by Al Hicks, NREL
Contact: Paul King
We have developed a robust, thermodynamic framework to understand key features responsible for electron bifurcation activity. We investigate the atomistic and molecular determinants of the redox cofactors and the protein framework that enable the strict coupling of the low- and high-energy electron transfer pathways with high fidelity, using a variety of experimental methods including ultrafast transient absorption visible spectroscopy, (spectro)electrochemistry, and electron paramagnetic resonance spectroscopy. We aim to develop comprehensive understanding of the thermodynamic and kinetic determinants of how these enzymes synchronize the spatial and temporal flux of electrons (and protons) to generate a high-energy product from starting materials of lower energy—the reward being the translation of this knowledge to real world applications.
Contact: Cara Lubner
Advanced Spin Resonance Facility
Integral to our research activities is the Advanced Spin Resonsance Facility at NREL.
Significance and Impacts
NREL's electron transfer research advances an understanding of how thermodynamics and kinetics of transfer reactions are controlled, the knowledge of which can improve the engineering of biological systems for biotechnological applications.
Contact
Share